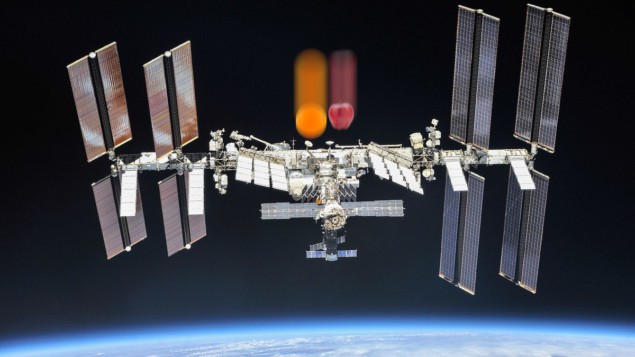
The motion of freely-falling bodies is independent of their composition. This is one of the foundations of Einstein’s Equivalence Principle (EEP), which underpins our modern understanding of gravity. This principle, however, is under constant scrutiny. Any violations of it would give us hints in our search for dark energy and dark matter, while also guiding our understanding of black holes and other systems where gravity and quantum mechanics meet.
Scientists from the US, France and Germany have now created a new system for testing the EEP: a mixture of two ultracold quantum gases that orbits the Earth aboard the International Space Station (ISS). They also demonstrated the first dual-species atom interferometer in space, which they describe as an “important step” towards testing the EEP. The question they aim to answer with this experiment is simple: do two atoms of different masses fall at the same rate?
Cold atoms on the ISS
The ISS is home to the Cold Atom Laboratory (CAL), which is a “playground” for atoms in space. Launched in 2018, in 2020 it created the first space-borne Bose-Einstein Condensate (BEC) – a special state of matter achieved after cooling atoms to temperatures just above absolute zero. This first quantum gas consisted of ultracold rubidium atoms, but following an upgrade in 2021, the CAL also hosts a microwave source for making quantum gases of potassium atoms.
In the latest work, which is described in Nature, the CAL scientists generated a quantum mixture of both species on the ISS. “Generating this quantum mixture in space is an important step towards developing high precision measurements for testing Einstein’s equivalence principle,” says Gabriel Müller, a PhD student at Leibniz University in Hannover, Germany who is involved in the experiment.
To achieve this mixture, the team confined rubidium atoms in a magnetic trap and allowed the most energetic “hot” atoms to evaporate out of the trap, leaving the “cold” atoms behind. This eventually leads to a phase transition into a quantum gas once the atoms drop below a certain critical temperature.
While this process also works for potassium atoms, simultaneously evaporating both species in the same trap is not straightforward. As the internal energy structure of rubidium and potassium atoms is different, their initial temperatures in the trap vary, and so will the optimum conditions of the trap and the evaporation time needed to reach the critical temperature. As a result, the scientists had to turn to a different solution. “The potassium quantum gas is not generated via evaporative cooling, but rather cooled ‘sympathetically’ via direct thermal contact with the evaporated ultracold rubidium gas,” explains Müller.
Generating this quantum gas in space has its merits, he adds. “On Earth, there’s a gravitational sag, meaning that two atoms of different masses will not be at the same position in the trap. In space, on the other hand, the gravitational interaction is weak, and the two species are overlapped.” This aspect of working in microgravity is essential for performing experiments aimed at observing interactions between the two species that would otherwise be hijacked by the effects of gravity on Earth.
The crucial role of quantum state engineering
Producing a quantum mixture of rubidium and potassium atoms brings the CAL team a step closer to testing the EEP, but other elements of the experiment still need to be tamed. For example, although the two species overlap in the trap, when they are released from it, their initial positions are slightly different. Müller explains that this is partly due to the properties of each atom species leading to different dynamics, but it is also due to the trap release not being instantaneous, meaning that one of the species experiences a residual magnetic force relative to the other. Such systematic effects could easily present themselves as a violation of the EEP if not taken care of properly.
For this reason, the scientists have turned their attention towards characterizing the systematics of their trap and reducing unwanted noise. “This is work that is actively being done in Hannover, to create well-engineered input states of both species, which will be crucial as you need similar initial conditions before you start the interferometer,” says Müller. One solution to the initial position problem, he adds, would be to slowly transport both species to a single position before switching off the magnetic trap. While this can be done with high precision, it comes at the expense of heating up the atoms and losing some of them. The scientists therefore hope to use machine learning to optimize the transport mechanism and thereby achieve similar control of the atomic dynamics, but much faster.

Dual-species atom interferometer in space
Once these problems are resolved, the next step would be to perform an EEP test using dual-species atom interferometry. This involves using light pulses to create a coherent superposition of the two ultracold atom clouds, then recombining them and letting them interfere after a certain free evolution time. The interference pattern contains valuable information about the mixture’s acceleration, from which the scientists can extract whether both species experienced the same gravitational acceleration.
A limiting factor in this technique is how well the positions of the laser beam and the atomic sample overlap. “This is the most tricky part,” Müller stresses. One problem is that vibrations on the ISS cause the laser system to vibrate, introducing phase noise into the system. Another issue is that the different mass and atomic energy level structure of both species leads them to respond differently to the vibrational noise, producing a dephasing between the two atom interferometers.
In the latest work, the scientists demonstrated simultaneous atom interferometry of the mixture and measured a relative phase between the interference pattern of the rubidium and the potassium atoms. However, they are well aware that such a phase is likely due to the noise sources they are tackling, rather than a violation of the EPP.
Future missions
A new science module was launched to the ISS with the goal of increasing atom number, improving the laser sources and implementing new algorithms in the experimental sequence. Fundamentally, though, the CAL scientists are striving to demonstrate inertial precision measurement at beyond the current state of the art. “Such realizations are important milestones towards future satellite missions testing the universality of free fall to unprecedented levels,” says Hannover’s Naceur Gaaloul, a co-author of the recent paper.
Bose–Einstein condensate is made onboard the International Space Station
One example Gaaloul mentions is the STE-QUEST (Space-Time Explorer and Quantum Equivalence Principle Space Test) proposal, which would be sensitive to differences in acceleration of as little as 10−17 m/s2. This precision is equivalent to dropping an apple and an orange and measuring, after one second, the difference in their position to within the radius of a proton. Space is, famously, hard, but atom interferometry in space is even harder.
- SEO Powered Content & PR Distribution. Get Amplified Today.
- PlatoData.Network Vertical Generative Ai. Empower Yourself. Access Here.
- PlatoAiStream. Web3 Intelligence. Knowledge Amplified. Access Here.
- PlatoESG. Carbon, CleanTech, Energy, Environment, Solar, Waste Management. Access Here.
- PlatoHealth. Biotech and Clinical Trials Intelligence. Access Here.
- Source: https://physicsworld.com/a/space-borne-atoms-herald-new-tests-of-einsteins-equivalence-principle/