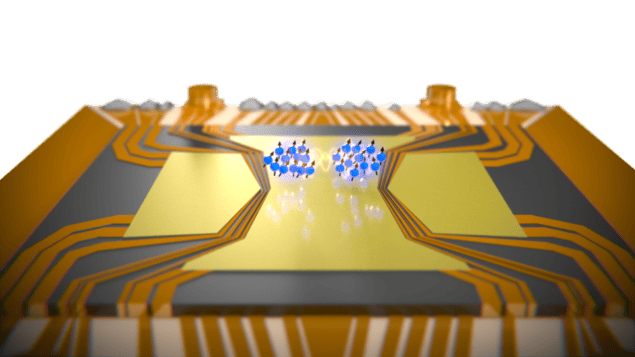
A key feature of quantum mechanics is the Heisenberg uncertainty principle, which states that complementary observables (such as position and momentum or two orthogonal components of a system’s spin) cannot be measured simultaneously with exact precision. In a famous 1935 paper, however, Albert Einstein, Boris Podolsky and Nathan Rosen (EPR) noted that if a quantum system is entangled with another auxiliary system far away, then a measurement made on the latter can be used to infer properties of the former. This would lower the uncertainty for the system’s observables below the Heisenberg limit – and, they argued, create a paradoxical situation that clashes with classical notions of realism and locality.
Since 1935, EPR’s paradoxical thought experiment has been performed numerous times with small, few-body quantum systems. However, the question of whether the non-classical character of quantum mechanics can be observed in larger systems had remained open until recently, when researchers from the University of Basel, Switzerland performed an EPR experiment for the first time in many-body quantum systems. Their results, which they describe in Physical Review X, confirm that quantum spookiness survives even when the number of quantum particles exceeds 1000.
Realizing the EPR paradox
The key to realizing an EPR experiment is to generate, preserve and verify strongly entangled states called EPR entangled states. Since entanglement is sensitive to noise, creating an interaction-free environment is crucial. To this end, Paolo Colciaghi, Yifan Li and co-workers used neutral rubidium-87 atoms that have been cooled to just above absolute zero and couple very weakly to their environment. These atoms can exist in two different quantum states, or levels, and thus behave like pseudo-spin 1/2 particles. The difference between the number of atoms in the two levels therefore provides a notion of the net spin of the atomic cloud.
The team began by applying an entanglement-generating interaction to this ensemble, thereby generating strong correlations among the atoms in the cloud. Next came the game changer that sets this experiment apart from other EPR realizations conducted on few-body quantum systems. By applying an interaction to half the atoms – an interaction that only affects the atoms’ external degree of freedom without disturbing their entanglement – the researchers coherently split the massive atomic cloud into two spatially separated entangled mesoscopic subsystems, A and B, each containing around 700 atoms.
The conflict: quantum vs classical
Once the subsystems were spatially separated, Colciaghi, Li and colleagues performed independent, local spin measurements on each atomic cloud. Firstly, they showed that over several hundred runs, random measurement outcomes of the spin components of clouds A and B were highly correlated. The team used these correlations to infer and improve predictions of the subsystem A’s properties locally. Consequently, they demonstrated that the product of the errors in the local measurement of A goes below the Heisenberg limit, thereby confirming the EPR paradox at a mesoscopic scale. “The non-classical character of quantum mechanics does not disappear when the system size is increased to this level,” says Colciaghi.
The team then went a step further, devising a set-up wherein simultaneous measurements of spin components could be made along different directions in the two clouds. As a special case, the Basel researchers measured a spin component of system A while simultaneously measuring the orthogonal spin of system B. They used the measurement at B to infer the orthogonal spin of system A. If EPR’s preferred theory of local realism were true, the measurement at B could not have affected system A due to the spatial separation between them. The inferred value of system A’s spin would thus be its definitive value, one that would exist even if no measurement was made there.
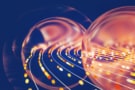
Bohr, Einstein and Bell: what the 2022 Nobel Prize for Physics tells us about quantum mechanics
This prediction of both orthogonal spins, simultaneously and with full precision, seemingly breaks the Heisenberg limit – but only if local realism is true. According to quantum mechanics, however, local realism does not hold. Local measurements can, in fact, affect spatially separated systems, and making a measurement of a quantum mechanical system collapses the system’s state irreversibly. Thus, the measurement for the orthogonal spin of A could only be inferred and not verified since the state of system A had collapsed, thereby losing all information about the state it was in.
Commenting on the results, Colciaghi tells Physics World that the observation of the EPR paradox in systems of more than 1000 particles confirms that either the completeness of quantum mechanics or the classical principle of local realism (or both) have to be given up. He adds that this experimental demonstration of many-body EPR entanglement of two spatially separated, individually addressable systems paves the way for designing systems that use entanglement as a resource, for example in quantum metrology. The discovery thus has technological as well as fundamental applications for quantum physics.
- SEO Powered Content & PR Distribution. Get Amplified Today.
- PlatoData.Network Vertical Generative Ai. Empower Yourself. Access Here.
- PlatoAiStream. Web3 Intelligence. Knowledge Amplified. Access Here.
- PlatoESG. Automotive / EVs, Carbon, CleanTech, Energy, Environment, Solar, Waste Management. Access Here.
- BlockOffsets. Modernizing Environmental Offset Ownership. Access Here.
- Source: https://physicsworld.com/a/einstein-podolsky-rosen-paradox-gets-a-1000-atom-test/