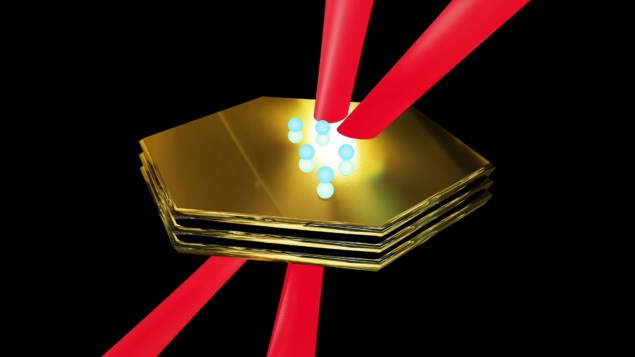
Gold has long been a popular way of enhancing the photosensitivity of electronic devices such as biosensors, imaging systems, energy harvesters and information processors. So far, the gold used has been polycrystalline, but in the past few years various research groups have finessed techniques for producing monocrystalline gold.
Researchers led by Anatoly Zayats at King’s College London, UK and Giulia Tagliabue at École Polytechnique Fédérale de Lausanne in Switzerland are now reporting that electrons in these new monocrystalline gold films behave significantly differently from electrons in polycrystalline gold. “We had surprises that we did not expect,” Zayats tells Physics World. The differences, he adds, could bring significant benefits for applications.
Plasmonic practicalities
Gold makes a useful photosensitizer because it supports a resonant response in which the oscillating electromagnetic field of the incident light makes electrons slosh back and forth collectively. This collective motion is termed a plasmon, and as the oscillation comes out of phase, energy in the plasmon passes on to electrons and positively-charged holes in the gold. Thanks to this transfer of energy, the electrons develop an effective temperature much higher than the material’s equilibrium temperature. It is these “hot” electrons that are so useful at initiating chemical reactions, signalling photon detection, stashing energy and so on. The main challenge is to extract them before they lose their energy.
For the most part, gold films are produced by sputtering the material onto a substrate, producing polycrystalline microstructures. Although the chemical processes required to grow monocrystalline gold have been known for some time, Zayats points out that “there is nothing for free in this world”, and the trade-offs are steep. Notably, for monocrystalline gold layers less than 100 nm thick, the maximum lateral dimensions are just a few micrometres, which restricts applications.
In the past couple of years, however, chemical processes have improved to the point that microflakes spanning hundreds of micrometres with less than 20 nm thickness are possible. These improvements prompted Zayats and his collaborators to explore what advantages they might have for plasmonic applications.
Double whammy
To investigate the possible benefits of monocrystalline gold microflakes, Zayats and his colleagues compared polycrystalline and monocrystalline versions using pump and probe pulses spaced just femtoseconds apart. These pulses enabled them to monitor the ultrafast decay processes of the hot electrons. They found that the electrons stayed hot much longer in the monocrystalline flakes, whereas in the polycrystalline flakes, the presence of grain boundaries led to more electron scattering and greater energy loss.
The researchers also found they could extract hot electrons much more efficiently from monocrystalline gold. Because the angle for total internal reflection of an electron incident on a gold surface is small, the surface of polycrystalline gold is deliberately roughened to increase the chances that an electron will hit the surface at an angle that allows it to escape and be extracted. In contrast, the surface of the monocrystalline gold was atomically smooth, yet the efficiency of electron extraction was close to the theoretical limit of 9%. The researchers attribute this to the longer hot electron lifetime, which means that the electrons have so many more encounters with the surface in a highly energetic state that they will eventually escape.
In contrast, Zayats notes that polycrystalline films take a double hit. “The energy of the electrons is lower and extraction efficiency is lower,” he says. When they began their experiments to compare the polycrystalline and monocrystalline flakes, he adds, it was not at all clear these effects would be so striking. Indeed, some of the team questioned the point of carrying out the experiments at all.
Fundamental differences
The study also revealed more nuanced differences. For instance, the researchers were able to detect the effects of the evanescent distribution of electrons that blurs the interfaces of materials, removing the sharp boundaries that appear in simple “toy” models. These evanescent electrons interact with phonons – lattice vibrations – in the adjacent substrate material. For thinner gold films these evanescent electrons make up a larger proportion of the electrons in the gold film, so the electrons overall lose their energy faster. However, the reverse is the case when the excitation laser power is ramped up because they are hotter and take more knocking around with phonons to cool down.
The results additionally indicated a change in band structure due to the longer-lived hot electrons. Although theory does suggest that mutual interactions between hot electrons and between hot electrons and lattice atoms might lead to this effect, it was not clear it would be noticeable at the moderate laser energies in the study. “You can imagine if you have high powers you start melting,” says Zayats. “To observe it at these low excitation powers, it was interesting.”
Gold nanorods are ideal candidates for long-term biosensing
Pan Wang, an optical engineer at Zhejiang University who was not directly involved in the study, describes it as “really impressive”. “These results are of great importance for a deeper fundamental understanding of non-equilibrium carrier dynamics in monocrystalline metals and provide a useful guideline for designing high-performance hot-carrier devices,” he tells Physics World. Referring to recent work showing that such films can be made even thinner, he adds that it would also be “very interesting” to investigate ultrafast carrier dynamics in nanometre-thick monocrystalline gold.
The results appear in Nature Communications.
- SEO Powered Content & PR Distribution. Get Amplified Today.
- PlatoData.Network Vertical Generative Ai. Empower Yourself. Access Here.
- PlatoAiStream. Web3 Intelligence. Knowledge Amplified. Access Here.
- PlatoESG. Carbon, CleanTech, Energy, Environment, Solar, Waste Management. Access Here.
- PlatoHealth. Biotech and Clinical Trials Intelligence. Access Here.
- Source: https://physicsworld.com/a/monocrystalline-gold-brings-electronic-devices-near-the-efficiency-limit/